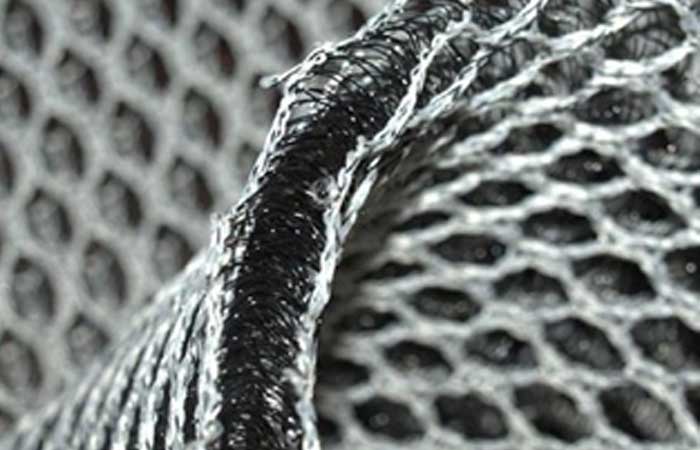
Innovating Spacer Fabric Technology
3D woven and knitted materials with complex structures have been developed to extend their applicability in functional and technical clothing by providing multifunctional properties.
The textile industry is currently experiencing heightened competition, prompting manufacturers to prioritise innovation and specialisation to establish a distinct presence in the
market. They are developing products that exceed the current performance and style ranges to appeal to the end-user market. Recent advancements in 3D textiles have led to the creation of novel fabrics and applications, highlighting the latest progress in 3D fibrous structures. 3D textiles offer numerous benefits, including reduced material consumption, a consistent yarn architecture, and fewer production steps. However, they reduce productivity by 5-10 times compared to regular 2D fabric production, increasing machine investment and process costs owing to complex parts and demanding machine handling.
Consequently, significant efforts have been made in recent decades to innovate and develop new varieties of 3D fabrics tailored for diverse technical applications. One such endeavour involves the creation of 3D spacer fabrics in woven and knitted warps and wefts with necessary modifications in the design of the machinery as well as in manufacturing technology. Spacer fabrics offer many attributes and characteristics that have not been achieved using other technologies. They offer a wide spectrum of properties, such as low area densities, low bulk densities, a wide range of thicknesses from 2 to 65 mm, high comfort or thermo-physiological properties, and tailor-made tensile, elastic, compression, and permeability properties, without using foam, rubber, latex, or other coating or laminating technique.
Spacer fabrics can be woven, nonwoven, braided, or knitted. Of these, woven and knit spacer fabrics are the most prominent and have many varied applications in technical textiles. Woven spacer fabrics are a specialised type of multi layered fabric in which two outer layers are formed using two independent warp systems with a third warp system that moves through the outside layers to form a 3D-spaced fabric. They have a variety of applications including filtration, cushioning, inflatables, and heat wicking. While standard weaving machines can be used for woven spacer fabrics with a small distance between the top and bottom layers, specialised machinery is required for fabrics with a significant distance between layers.
However, 3D spacer-knitted fabrics have been widely used in the engineering field because of their smooth and efficient processing in both warp and weft knitting. Knitted spacer fabrics can be produced using a flat knitting machine, two-needle bar raschel knitting machine (warp-knitted spacer fabric), and circular double jersey machine (weft knitted spacer fabrics). Three-layer weft-knitted spacer fabrics have a structure consisting of two separate knitted layers joined by a connective yarn. Such materials may have the same or completely different structures in the face and back layers and different types of yarns used in the connecting layer.
Owing to their characteristics, spacer fabrics exhibit different properties that cannot be met by conventional textiles and cannot be achieved using other technologies. These fabrics continue to find new and novel product applications in various fields, such as protective clothing, transportation, geo-textiles, buildings, packing materials, military equipment, medicine, and sports. In the realm of technical textiles, where the market has become saturated and international competition in the clothing, home, and household textile sectors is fierce, the textile industry is becoming increasingly interested in new technologies. The innovative weaving and knitting of 3D fabrics represent an ideal platform for synthesising spacer fabrics with unique properties, applications, and a special product group within the innovative functional textile sector.
It is generally recognised that spacer fabrics will be extensively used in a wide range of new products, especially for niche applications where their aesthetic, functional, and technical properties are a big advantage. 3D woven and knitted materials with complex structures have been developed to extend their applicability in functional and technical clothing by providing multifunctional properties. However, the lack of a critical review on innovative spacer fabrics, especially for technical textile applications, is a sound basis for this review. Hence, a review is compiled describing the innovation of 3D woven and knitted spacer fabrics and the recent applications of these spacer fabrics in technical textiles. This paper discusses the advances in 3D woven and knitted spacer fabric behaviour for technical textile applications.
Production technology of weft knitted spacer fabrics
Spacer fabric knitting in dial and cylinder machine. WeKSFs, such as double jerseys, can be created on circular dial and cylinder machines using various stitch combinations to connect the two fabric layers. Ray stated that all techniques necessitate the use of at least three yarns for each visual fabric course: cylinder needle yarn, dial needle yarn, and a spacer yarn, typically monofilament. Dial height adjustment can manipulate the distance between the two fabrics and determine the amount of pile yarn. Techniques, such as tucking and plating, are used to create spacer fabrics in cylinders and dial machines. Therefore, special feeders may be necessary for this purpose. Jacquard patterning can also be used in dial and cylinder machines for creating spacer fabric effects. However, the production of spacer fabrics on circular knitting machines faces technical limitations owing to the geometry of the yarn feeding path of the knitting head. The thicker the fabric, the higher the dial and dial cap that must be raised, resulting in more acute yarn feeding angles.
Spacer fabric knitting in a flat knitting machine. Flat-bed knitting machines offer high flexibility for producing 3D knitted textiles of various shapes, including spacer fabrics, near-end shape textiles, and tubes. This allows for the production of near net-shaped fabrics, eliminating most manufacturing processes, and achieving a zero-waste approach in production. Flat knitting machines can be used to shape textiles by varying the number of stitches in the wale and/or the course direction and stitch length. In particular, techniques such as widening, narrowing, bind-off, and partial knitting are used for shaping. Widening activates additional needles at the edges, whereas narrowing deactivates them. Loops from the outer needles were transferred to adjacent needles, tailoring the edge of the fabric to the desired width.
The bind-off creates a fixed edge at the end of the knitted fabric. These techniques can be combined to create a near-net shape textile. A flat-bed knitting machine can produce two types of 3D spacer fabrics: conventional spacer fabric and innovative spacer fabric. Knitting of conventional WeKSFs. AV-bed weft knitting machine produces a knit fabric on two straight rows of hook-shaped needles. Spacer fabric knitting involves two basic steps: forming face rows using knit operation sequences and adding filler rows to the same needles using the tuck operation, as shown in Figure 1(a). Second, the filler yarn formed a shallow lengthwise zigzag by tucking at regular intervals onto alternating beds, as shown in Figure 1(b).
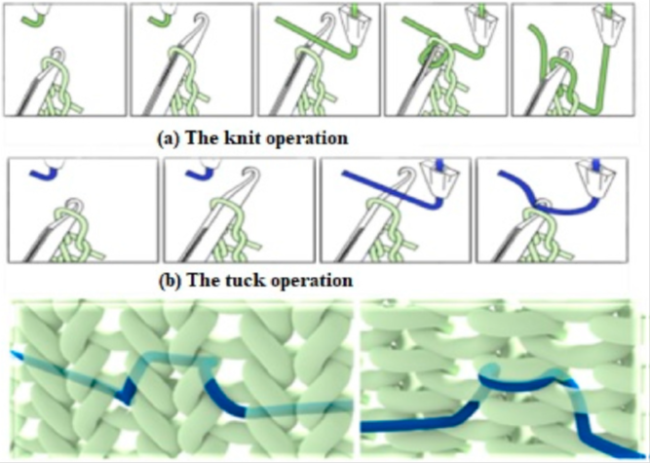
Figure 1: The V-bed 3D spacer knit and tucks operations.
This process separates the two faces of the fabric by using only a small gap between the two needle beds. Subsequent passes of filler yarn add density to the same face row of the fabric because tucking does not add height to the fabric. The faces are knitted with tension-sensitive elastic yarn,
which later shrinks, pulling the filler yarn in and pushing them apart into the spacer fabric’s characteristic “fluffy” thickness, resulting in a unique and comfortable design. Reinforcement yarns are integrated into flat-knitted 3D spacer fabrics to improve their tensile properties.
These yarns can be replaced with functional or conductive yarns, such as carbon filament copper wires or other high-functioning fibres. This process allows these fabrics to be used as high-functioning fabrics, thereby enhancing their durability and performance. The process of integrating reinforcement in the spacer fabric involves knitting a single jersey on the front and back needle beds (A1, A2) until the height of requirements (H) P1 and P2 is reached (a). The float is knitted separately on the front and back needle beds, and a tuck can be placed after the interval length to prevent the float line from being too long (b). A single jersey was knitted individually on both needle beds (L1 and L2) until half of the required height was achieved (c). The connection with the rib courses was performed by needle selection using the same yarns (d). The jersey is then knitted again individually on both needle beds (L3 and L4), returning to P1 and P2 (e), as shown in Figure 2.
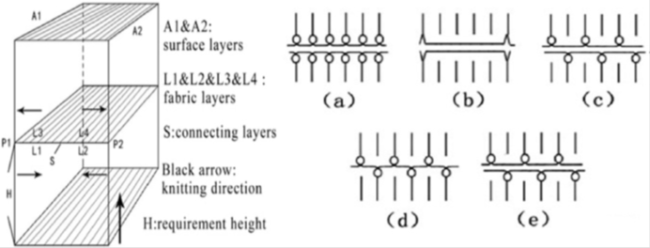
Figure 2: Knitting flowchart of 3D spacer fabrics with reinforcement yarns
Knitting of innovative WeKSFs. The computerised flat-knitting technique offers innovative structures using unique technical features such as sinkers, transfer, and racking. Spacer fabrics feature complex three-dimensional (3D) constructions with two layers bonded together. However, yarns must travel long distances from the yarn feeder to the knitting area, which causes significant friction and input tension.
Therefore, the simplified yarn path in Figure 3 is crucial for smooth knitting, and turning down the comb pull is beneficial for narrow knitting areas, highlighting the technological basis for manufacturing innovative 3D spacer fabrics. These innovative spacer fabrics include 3D spacer fabrics without any reinforcements, 3D curvilinear knitted spacer fabrics, and 3D tubular spacer fabrics. 3D spacer fabrics consisting of multilayer reinforced surfaces and connecting layers were initially developed without reinforcement using innovative knitting techniques. Once successful, the necessary reinforcements can be achieved through further developments in flat knitting technology using advanced integration concepts to achieve the necessary reinforcements in the course and wale directions, as shown in Figure 4(a).
However, spacer fabrics have been developed by creating individual planes and connecting layers composed of single jersey structures. The flat-knitting technique for 3D spacer fabrics allows for the creation of curvilinear shapes in the warp direction, a novel structure that can be developed from flat-knitted 3D spacer fabrics. Hamedi et al. focused on creating weft-knitted spacer fabrics with U-shaped geometry chosen for their designable structures, as shown in Figure 4(b) They used polyester yarns with a 1680 denier for knitting on a computerised flat knitting machine (Stoll CMS 400; E5).
Weft and warp 3D knitting technologies produce single, bifurcated, multi branched, seamless tubular structures. Circular weft machines create single-layer tubes, whereas flat-bed weft knitting machines produce complex tubular structures.
Various designs can be created using a combination of materials and stitches, such as plain plating, to create artificial tracheal stents. This knitting structure consists of
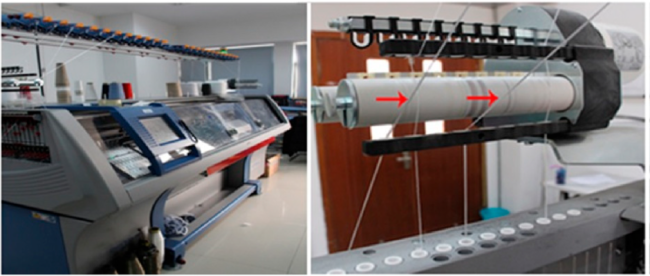
Figure 3: STOLL computerised V-bed knitting machine with simplified yarn path
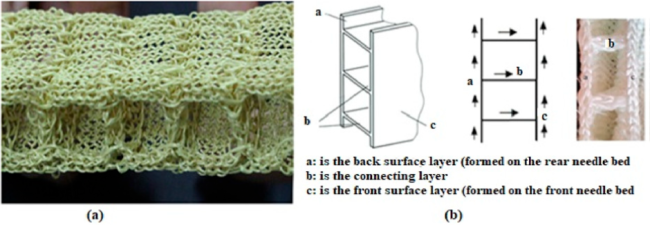
Figure 4: (a) Flat-knitted 3D spacer fabric without reinforced yarns44; (b) Typical schematic and real configuration of the U-shaped 3D weft-knitted spacer fabric sample
two or more yarns with different features that influence their morphology and physical properties. Furthermore, advanced knitting of the base 3D spacer fabric from the conductive silver-plated nylon fibres (SPNFs) SHIMA SEIKI SVR123SP-14G computerised knitting machine was adopted to knit the 3D spacer fabric. The flat knitting machine mainly consisted of a carriage and two horizontally positioned needle beds. The fabric was created using two sets of conductive SPNFs and two sets of dielectric polyester fibres via computerised knitting programming, as shown in Figure 5. The computerised fabrication process involves three steps: knitting two sets of conductive SPNFs to the top and bottom layers using the intarsia technique, inserting a spacing layer using inlay knitting to avoid short-cutting two conductive layers, and using one set of polyester fibres to knit the left and right edges using interlock and tuck stitches
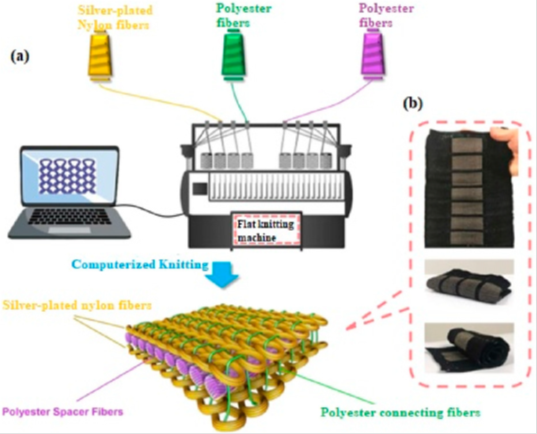
Figure 5: Conductive 3D weft knitted spacer fabric; (a) production process, (b) product
Warp-knitted spacer fabrics
WKSFs are structures consisting of two fabric layers joined back-to-back, made from different materials, and with different structures. Yarns joining the layers can either fix them directly or space them apart. WKSFs are unique structures with 3D space, typically ranging from 1 to 15mmthick, with two faces from 0.4 to 1mmthick. The thickness depends on the gap between the needle beds, yarn type, and the base fabric structure. Spacers can vary in structure from inelastic to elastic solid, net, or textured surfaces. Stability is controlled by the threading and lapping of the guide bars, ensuring both length- and width-wise stability. The compression and resilience properties of the spacer can be adjusted based on the guide bar material and the lapping pattern. The fabric can be used in its original form or separated using a pile shearing machine to create a plush or velvet fabric by slitting it in the middle. Warp knitting technology offers high pattern variation, allowing for the precise adjustment of pressure stability and air permeability in spacer fabrics. These fabrics are commonly used in seating, automotive, functional clothing, mattresses, medicine, support textiles, and orthopedics. Their sideways open structure provides elasticity, insulation, and acoustic damping properties and is also used for filtration and water harvesting methods . Hu et al. highlight the advantages of 3DWKSFs, including their affordability, ease of manufacturing, improved handling of preform layers, better impact damage tolerance, delamination resistance to loads, inter laminar fatigue resistance, and enhanced joint strength under monotonic and cyclic loading.
Knitting of WKSF on raschel machine
Karl Mayer is a leading manufacturer of warp knitting machines with double-needle beds for producing spacer fabrics, including the double needle bar raschel machine with six guide bars. Figure 6 shows that bars 1, 2, and 5, 6, respectively, are used to knit the front and back surface layers.
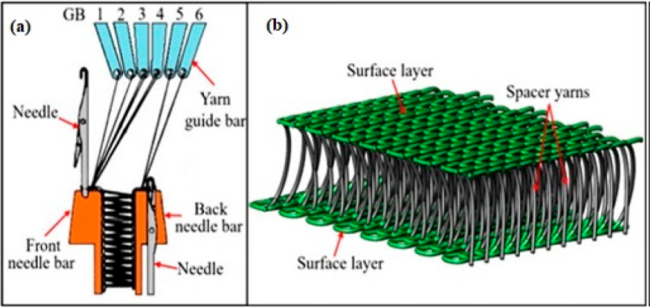
Figure 6: The (a) working principle of the double-needle-bar warp-knitting machine and (b) schematic illustration of warp-knitted spacer fabric
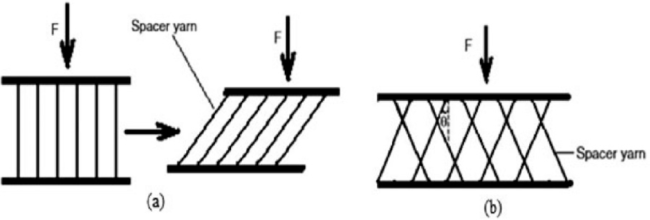
Figure 7: Connection with vertical spacer yarns and (b) Connection with inclined spacer
yarns
To connect the two layers, bars three and four feed the spacer yarn on both needles, typically making symmetrical lapping movements for a better connection. Two commonly used methods for connecting the two surface layers are shown in Figure 6.
Figure 6(a) shows two surface layers connected by vertical spacer yarns; however, this structure is unstable because of the horizontal inclination of the yarns under pressure. The stability of the structure was enhanced by connecting two surface layers with symmetrically inclined spacer yarns, as shown in Figure 6(b). The inclination angles of these yarns can be adjusted by changing the underlap amounts to satisfy different end-use requirements. A larger underlap resulted in a higher spacer yarn inclination.
Furthermore, jacquard double-bar Raschel machines can produce tubular structures and spacer fabrics, including 3D non-crimp fabrics based on warp-knitted spacer fabrics. This requires the integration of warp yarns and weft yarns, and requires a double-needle bar raschel machine with a double-faced weft insertion system. High-performance yarns, such as glass yarns, can be integrated on both sides in 0° and 90°directions (Figure 7), enabling the production of open textile-reinforced spacer warp-knit mesh structures for concrete applications.
Warp knitted spacer fabric variation by process modification
Machine modifications are needed to create more complex spacer fabrics with additional spatial curvatures using double-needle bar warp knitting technology. Modifications in the double-needle bar warp knitting process can be achieved by adjusting the trick plate spacing and position, fabric take-off and yarn tension, and integrating additional technologies. Figure 8 shows a textile semi-finished product for fibre reinforced plastic profiles based on Arnold and Hufnagl’s patent. These structures are primarily used to produce T–or double-T profiles. The bar was made from a right right multiaxial warp knitted structure, while the legs were made from right-left multiaxial warp knits. The spacer fabric was fabricated by adjusting the spacing and position of the trick plates.
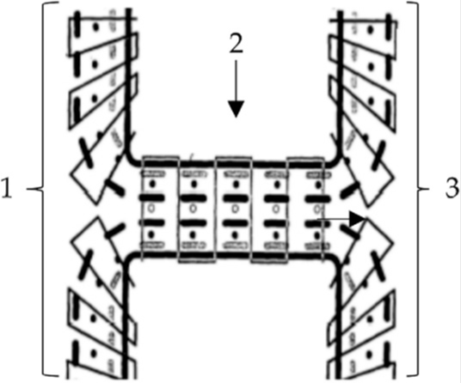
Figure 8: Textile semi-finished product for fibre-reinforced plastics (1, 3: legs made of right-Left multiaxial warp knits, 2: bar made of a right-right multiaxial warp knits
The double-needle bar warp knitting process can be further enhanced by using a knitted fabric take-down with conical rollers, resulting in arc-shaped closed reinforcing knitted fabrics with in-plane curvature. Advanced techniques also enable the production of spacer warp knits with alternating distances within the working width, creating contoured closed or lattice-like surfaces. Franz developed warp-knitted spacer panels and tubular WKSFs (Figure 9), which can be produced by varying the fabric takeoff and yarn tension. This technology allows for the development of fibre-reinforced lightweight components for large-area components, such as ceilings, walls, floors, and double-walled pipes. The spacer structures produced in integral design have a six-fold higher compressive strength compared to conventional structures like honeycombs and glass spacer fabrics.
Luling developed a lightweight building technology that combined 3D spacer fabrics with 3D printed objects, allowing for targeted shading and insulation. This technology focuses on using the same materials for both print and textile materials, making recycling easier. An example of this structure is shown in Figure 10.
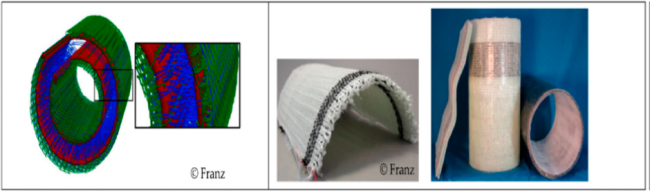
Figure 9: Tubular warp knitted spacer fabrics (left: simulation model, right: textile and consolidated demonstrator
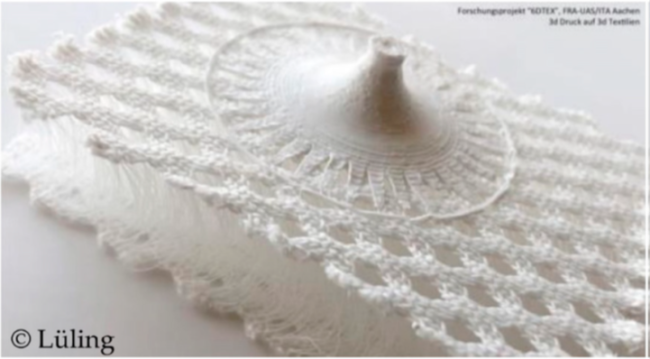
Figure 10 – WKSF combined with a 3D-printed parts for lightweight building
References
- Bekinew Kitaw Dejene1,2 and Adane Dagnaw Gudayu, Exploring the potential of 3D
- woven and knitted spacer fabrics in technical textiles: A critical review, Journal of Industrial Textiles, Volume 54: 1–55, 2024.
- N.Gokarneshan, Analysis of compression behaviour of warp knit spacer fabrics for evaluating suitability in cushioning applications, Journal of Textile, Apparel, Technology and Management, 2018.
- N.Gokarneshan and K.Velumani, Some significant advances in spacer fabric technology for newer areas of applications, Journal of Textile Science and Engineering, 2018.
- N.Gokarneshan and U.Datchayani, Advances in medical knits, Journal of Textile Engineering and Fashion Technology, 2017.
- N.Gokarneshan, Role of knit spacer fabrics in treatment of pressure ulcers, Global journal of addiction and rehabilitation medicine, 2018.
- N.Gokarneshan et.al., Technology of warp knit spacer fabrics, Woodhead publishing India in Textiles, 2020.
- N.Gokarneshan et.al., Application of warp knit spacer fabrics in Tissue Engineering, Journal of Textile Engineering and Fashion Technology,
- N.Gokarneshan et.al., Stab resistant warp knit spacer fabrics, International journal of applied engineering and technology, 2016.
- N.Gokarneshan et. al, A comparative study of the geometrical properties of knit fabrics produced from soya bean and cotton fibres, Man made textiles in india, 2011.
- N.Gokarneshan and K.Thangamani, A comparative evaluation of the low stress mechanical properties of cotton/spandex and polyester/spandex blend knits, International journal of applied engineering and technology, 2012.
- N.Gokarneshan and K.Thangamani, Evaluation of the mechanical properties of core and plated spandex blend knits, 2013.
- N.Gokarneshan and G.Nagarajan, Knitting technology for producing advanced composite preforms, Asian Textile Journal, 2005.
- N.Gokarneshan, Emerging research trends in medical textiles, Springer Nature, 2015.
About the author:
- Sona.M.Anton, R Sasirekha, Z.Shahanaz, are from the Department of Fashion Design and Arts, Hindustan Institute of Technology and Science, Chennai, Tamil Nadu.
- Dr N Gokarneshanis from the Department of Textile Chemistry, SSM College of Engineering, Komarapalayam, Tamil Nadu.
- Dr C Kayalvizhiis from the Department of Textile Technology, Jaya Engineering College, Tiruninravur, Tamil Nadu.
- Dr U Ratnais from the Department of Textiles and Clothing, Avinashilingam Institute of Home Science and Higher Education for Women, Coimbatore, Tamil Nadu.
- K Velumani, S P Thangavel and M Tamilanbanis from the Department of Textile Technology, SSM College of Engineering, Komarapalayam, Tamil Nadu.
- S R Viswanathis from the Department of Textile Technology, Park College of Engineering and Tekhnology, Coimbatore, Tamil Nadu.