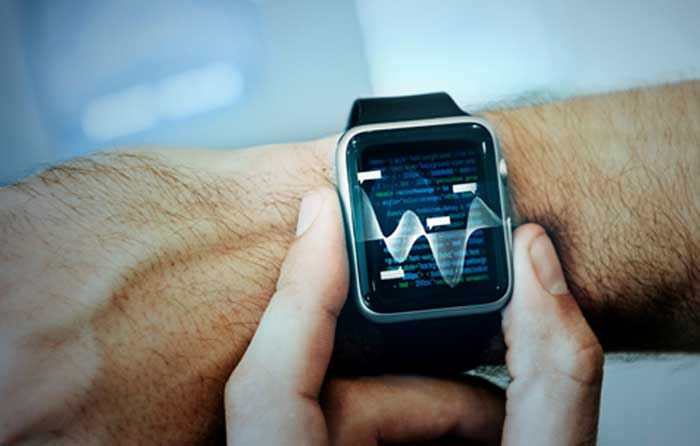
Role of smart textiles in heart monitoring
As textile clothing is one of the most frequently used materials in our daily life, they are ideal for wearable health monitoring systems.
With the rapid development in technology and the ever-increasing demands of people, conventional textiles are becoming inadequate for our uses. Traditionally, textile clothing is expected to have a good fit, comfort, and durability for use. Nowadays, these requirements are not enough due to growing competition on the market and changes in society demand supported with technological advancements. These societal demands and technological advancements led to the development of “smart textiles.” Smart textiles are defined as materials that are able to change their behavior as a response to the influence of external factors or stimuli from the surrounding environment such as from mechanical, thermal, chemical, electrical, magnetic, or other sources. Based on their level of “smartness”, smart textiles can be categorised into three subgroups: passive, active and very smart.
Passive smart textiles: textiles that only sense the environmental condition, and react to the stimuli passively, e.g., biopotential sensors. Active smart textiles: textiles that are able to sense the stimuli from the environment and respond to that particular stimulus. This can be achieved by integrating an actuator function and a sensing device. An example is a temperature-aware shirt which will automatically roll up its sleeves when body temperature becomes elevated. Very smart or intelligent textiles: textiles that are able to sense the environmental stimuli, give reaction to the stimuli, and thirdly adapt their behaviour to the given circumstances. In the future, intelligent fabrics are expected to be integrated with cloud computing. For example, patients with a homecare medical device could send vital signals to their doctor to diagnose their health condition.
Smart textiles are commercially available in different applications such as in sports, healthcare, vehicle industry, military, personal protection, and safety and space exploration. Although smart textiles are used in all spheres of our lives, healthcare is the most remarkable market area, potentially enabling the development of new healthcare systems that can ensure significant cost reductions. Due to the increasing complication of medical treatments on the one hand and the advancement of technology in the area, on the other hand, there is an emerging trend for a personalised healthcare system. Smart textiles have the lion’s share in this regard, where uses are equipped with wearable sensors to monitor their vital signs continuously and they give greater potential to users to take active control of their health as part of a preventive lifestyle which brings a reduction in healthcare cost by the early detection of health problems.
Devices that monitor physiological activities such as heart activity or electrocardio-gram (ECG, also called EKG), brain activity/electroencephalogram, muscle activity/electromyography, and other health indicators such as skin temperature, respiration, breathing, sweating rate, etc. have tremendous advantages in
monitoring health. ECG is the process of recording the electrical activity of the heart, one of the most important physiological signals, which contains a treasure trove of information about the heart condition and heart-related diseases, such as arrhythmia, cardiac arrest, premature atrial contraction, premature ventricular contraction, congestive heart failure and coronary artery disease. Recently, wearable ECG devices that enable us to continuously monitor heart activity are being developed as textile-based devices. As textiles are an indispensable part of our life, this is quite convenient for handling. In the review paper by Pain et al., a survey on textile electrodes for ECG monitoring and the different materials used to develop textile electrodes, factors that affect their performance in the signal acquisition were presented.
This paper aimed to provide a scientific overview of textile-based electrodes for ECG monitoring, with the main emphasis on the different types of electrodes and recent advancements on ECG electrodes in general, and dry textile electrodes in particular. Developments in wearable health monitoring clothes and conductive textiles, especially for ECG monitoring, are also addressed.
Textile-based ECG electrodes
Wearable electronic smart textiles can be developed by using the textile fabric itself as a sensor or embedding the sensor in textile clothes. The integration of flexible ECG sensors with everyday textiles will be convenient for handling and cost-saving purposes.
Instead of attaching a separate electrode like a disposable Ag/AgCl electrode, making the textile itself a sensing electrode is more interesting for monitoring the health and wellbeing of individuals demanding long-term heart monitoring. Textile-based sensors could contribute possibilities for providing more affordable, accessible, and easy-to-wear measuring devices, thus giving a greater potential to users to take active control of their health as part of a preventive lifestyle which brings a reduction in healthcare cost by the early detection of health problems. However, conventional textile products that are found in everyday garments are intrinsically electrically non-conductive and hence cannot be directly used for bio-sensing applications. Sensing or data transmission via the textile material requires the textile to be electrically conductive. Textiles can be made electrically conductive by integrating metals, carbon materials, or conductive polymers into the textile structure through several techniques at different stages (fibres, yarns, or fabrics).
Wearable textile electrodes for continuous health monitoring products, as part of standard clothing, are needed to satisfy several requirements. The most important requirement is that they should have adequate electrical conductivity. Sufficient electrical conductivity is necessary to detect even small amplitudes in the electrophysiological signals of the heart. High electrical conductivity results in lower skin–electrode impedance which is very essential during ECG measurements to acquire high signal quality. Additionally, as part of standard clothes, textile electrodes should have a good visual appearance taking into account fashion aspects, and at the same time be comfortable for the wearer.
Furthermore, the conductive textiles should allow standard maintenance such as washing and ironing. In addition, the electrodes should be easy to wear and use, and should be as lightweight as possible, though should not hinder the users’ movement and daily activity. Apart from the electrodes, the signal recording also needs interconnections and data processing and possibly an antenna, which should also be integrated (completely or partly) into the garment. After data processing, the result may then in turn be used to display information on the health status of the wearer, either to the user or to the concerned people such as their physician. All these parameters of wearable sensors make designing such products very challenging as many conflicting requirements must be considered during product development.
Depending on the coupling between the electrode and the skin, textile-based dry electrodes can be categorised into two types: contact and non-contact electrodes. In contact electrodes, the direct physical coupling is established between the skin and the electrode. Electrodes are required to have continuous conformal contact with the skin of the wearer to allow consistent signal detection and to minimise artifacts and noise, i.e., unwanted signals. When electrodes are directly attached to the skin, they should be biocompatible not to cause any negative impact on the skin of the user, whereas in non-contact electrodes, there is no physical contact to the skin and the electrodes are rather separated from the skin by a dielectric material or air. In a non-contact system, the electrodes should be kept at a fixed distance from the skin for optimal operation. These sensors function by the principle of sensing the electric field created by the displacement currents in the body through the coupling of charges between the patient’s skin and the electrode and are called capacitively coupled electrodes. Non-contact electrodes are especially important when contact electrodes damage the skin like in newborns. Most electrodes used are contact electrodes and provide better signal quality. These textile- based electrodes can be developed by integrating yarns or wires to the textile structure or applying conducting compounds onto the textile fabric surface. In the following section, the methods to make textile electrodes for ECG applications have been covered in detail.
Design aspects
As an alternative to wet electrodes, dry textile electrodes that do not require gel electrolytes are suitable for wearable long-term biosensing to monitor cardiovascular diseases. Textile electrodes could be used in a wide variety of applications and have a larger contact area compared to other dry rigid non-textile electrodes, but when embedded in standard clothes, they often do not provide complete conformal contact with skin. Conductive textiles are created by coating a conductive component on the textile surface or by integrating metal fibres during the manufacturing stage, but because of this, the flexibility of the fabric is negatively impacted, and the fabric is no longer able to fit conformably with the curvilinear surface of the skin. For this reason, textile electrodes are usually integrated inside tight fit garments during the manufacturing stage or patches of textile electrodes are embedded inside elastic bands or tight fit shirts to keep the electrodes in their position and to ensure the proper connection to the skin of the user. However, nonetheless, the electrodes create minimal movement over the skin surface, which causes variation in skin–electrode impedance and negatively affects the signal quality by generating noise interferences. To improve skin–electrode contact, prevent displacement of the electrodes on the skin surface during body motion, and ensure uniform holding pressure, some researchers prepared textile electrodes in a sandwiched structure by sewing pieces of conductive fabric with a foam layer and an outer layer of non-conductive fabric, or simply sew the conductive fabric to a non-conductive synthetic leather. Moreover, slightly moisturising the skin or the surface of the textile electrode with a spray
of tap water (saline solution) helped to lower skin–electrode contact impedance and as a result, improved signal quality, which shows the skin moisture (sweat) would also help to improve signal quality. Textile is especially suited for this due to the capillary suction that can retain the water. Weder et al., developed a breast belt with an embroidered textile electrode together with a small water reservoir to keep the skin–electrode interface humid and to obtain good signal quality. However, keeping the skin moist for a long period may cause discomfort to the users. On the other hand, in, an ionic liquid gel is used to enhance continuous skin–electrode contact and to improve acquired signals using textile electrodes under dynamic conditions. Figure 1 presents some design examples of wearable textile ECG monitoring systems.
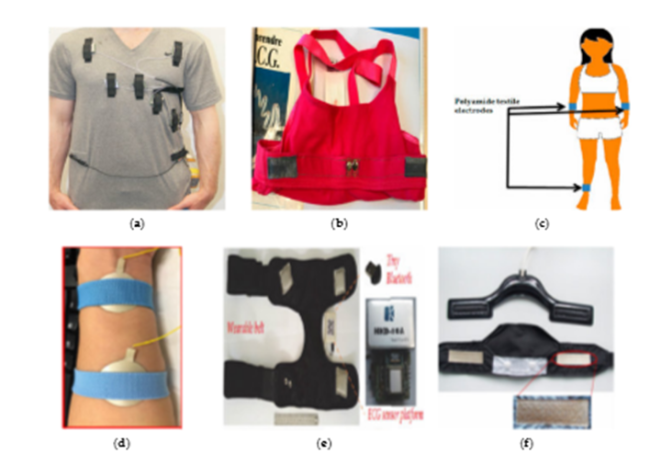
Figure 1: Different wearable textile ECG monitoring systems: (a) ECG T-shirt with active electrodes and connectors; (b) PEDOT:PSS-coated polyamide electrodes sewn into bras; (c) electrode placement for ECG measurement where plastic clamps were used to fix the electrodes onto the wrist; (d) ECG sensing wristband with printed and flexible electrodes; (e) wearable chest belt with silver-coated nylon woven electrodes and Bluetooth module (f) ECG belt with wetting pad (above) and the embroidered electrodes (below)
Research outputs prove that textile electrodes are full of motion artifacts because of an unstable contact of the electrodes on the skin leading to high skin–electrode contact impedance. The skin–electrode contact impedance depends on contact pressure, electrode placement, user activity and muscle activity in addition to the main textile electrode impedance. Similarly, currently available wearable health monitoring devices employ cables to connect electrodes to a data processing unit which hinders user activity and leads to discomfort. Due to this, they are not in routine clinical use yet. Designing a wearable ECG monitoring system that guarantees a permanent skin–electrode contact and allows high ECG signal quality, which would be important to develop a true textile wearable system. Additionally, the washability and the dimensional and environmental stability of the electrodes are key issues for long-term use that should be considered.
Generally, previous research in the area of textile-based electrodes has overcome several problems associated with wearable biopotential monitoring devices, however, the comfort of the user and the ECG signal quality is not at a satisfactory level. It is possible to overcome some of the drawbacks of textile electrodes through optimal design, such as higher contact pressure, moisturising, increasing electrode size, and others. However, many of these changes lead to other problems, mostly concerning the comfort of the user.
Future perspective
The demand for wearable health monitoring electronic textiles is promptly increasing due to their flexibility, lightweight, and washing advantages over standard electrodes. The use of textile-based electrodes to monitor physiological activities also avoids the use of gel to reduce skin-to-electrode impedance. Therefore, such electrodes would be an ideal replacement for the gel-based wet electrodes and are promising electrodes for wearable applications for long-term monitoring.
Since the introduction of textile-based ECG electrodes in the 1990s, the research in finding better electrodes has drastically increased with much promising improvement in artifact minimisation, impedance lowering, SNR minimisation, and signal quality improvements. For instance, searching with “ECG + textile” results in 316 Web of Science indexed articles and conference proceedings that have been published in 30 different refereed journals in the last three decades (1990–2020). Moreover, the publication rate is increasing as shown in Figure 10. This shows that many experts are spending their time in the lab looking for improved textile electrodes. This pace of research should eventually result in finding working, washable and textile electrodes for long-term monitoring.
The rapidly increasing demand for e-textiles for physiological monitoring resulted in new conductive materials investigations. New state-of-the-art integration techniques of electronic components in the textile structure could also evolve as a worthy solution in the near future. For instance, the use of 4D printing and investigating 4D conductive materials that are able to change their size and dimension under external controlled stimuli could play a role in lowering skin-to-electrode impedance and improving fit and design concepts.
Smart wearable textiles are the result of interdisciplinary research, which connects concepts and expertise from textile engineering, computer engineering, electronics, material science, medicine, and more. The integration of expertise and the high demand of markets for smart textiles leads to continuous improvements in the technology, supported by growth in research. The development of a reliable textile-based ECG electrode requires a collaboration of experts from textile, material, medical and electrical engineering.
Conclusions
The rise in health care costs and advancements in technology necessitates an emerging trend for personalised health care. Various electrical physiological functions can be observed by means of wearable health care devices. Of the different bio-signals, ECG signals are considered crucial since they offer information regarding the heart condition and cardiovascular diseases which is one of the most prevalent causes of death worldwide. For the early detection and diagnosis of cardiovascular diseases, the continuous and long-term measurement and monitoring of the ECG signal is very important. The commercially used Ag/AgCl gelled electrodes are not suitable for long-term wearable health monitoring systems, due to dehydration of the conductive wet gel over time which causes signal quality deterioration and discomfort for the user, and because they are directly attached to the skin with a connecting wire, often leading to discomfort at the electrode location over time. Dry electrodes are considered a good alternative to those gelled electrodes, especially for long-term use. Dry electrodes made of rigid metals and conductive textile products have been reported by many investigators.
Textile electrodes for ECG monitoring can be developed by integrating metal yarns into the textile or coating with metal nanoparticles, coating with carbon materials, and coating with conductive polymers. As textile clothing is one of the most frequently used materials in our daily life, they are ideal for wearable health monitoring systems. Wearable textile electrodes for continuous health monitoring products need to satisfy requirements such as high conductivity, aesthetics, and comfort, conformal skin–electrode contact, and biocompatibility. Although previous research on the area of textile-based electrodes overcame several problems associated with wearable biopotential monitoring devices, the comfort of the monitored person and ECG signal quality is still not at a satisfactory level.
Mostly, textile electrodes are prone to motion artifacts due to higher contact impedance and the absence of conformal contact, which is why they have not yet achieved acceptance for clinical standard use. The current review showed that there are still a lot of challenges to be resolved for textile electrodes and wearable smart textiles to become more applicable in real-life situations and also become accepted by patients and other users as a reliable, multifunctional, easy-to-use, and minimally obtrusive technology that can increase their quality of life.
References
- Tao, X. Smart technology for textiles and clothing—Introduction and overview. In Smart Fibres, Fabrics and Clothing; Woodhead Publishing: Cambridge, UK, 2001; pp. 1–6. ISBN 0849311721.
- Koncar, V. Introduction to smart textiles and their applications. In Smart Textiles and Their Applications; Koncar, V., Ed.; Woodhead Publishing: Cambridge, UK, 2016; pp. 1–8. ISBN 978-0-08-100583-5.
- Stoppa, M.; Chiolerio, A. Wearable Electronics and Smart Textiles: A Critical Review. Sensors 2014, 14, 11957–11992.
- Lee, Y.-D.; Chung, W.-Y. Wireless Sensor Network Based Wearable Smart Shirt for Ubiquitous Health and Activity Monitoring. Sens. Actuators B Chem. 2009, 140, 390–395.
- Wang, Y.; Deepu, C.J.; Lian, Y. A Computationally Efficient QRS Detection Algorithm for Wearable ECG Sensors. In Proceedings of the Annual International Conference of the IEEE Engineering in Medicine and Biology Society, EMBS, Boston, MA, USA, 30, August–3 September 2011; pp. 5641–5644.
- Das, S.C.; Chowdhury, N. Smart Textiles—New Possibilities in Textile Engineering. IOSR J. Polym. Text. Eng. 2014, 1, 1–3.
- Sun, F.; Yi, C.; Li, W.; Li, Y. A Wearable H-Shirt for Exercise ECG Monitoring and Individual Lactate Threshold Computing. Comput. Ind. 2017, 92, 1–11.
- Patel, S.; Park, H.; Bonato, P.; Chan, L.; Rodgers, M. A Review of Wearable Sensors and Systems with Application in Rehabilitation. J. Neuroeng. Rehabil. 2012, 9, 1–17.
- Drean, E.; Schacher, L.; Adolphe, D.; Bauer, F. Smart Textiles for Automotive: Application to Airbag Development. Exp. Tech. 2008, 32, 44–48.
- Scataglini, S.; Andreoni, G.; Gallant, J. Smart Clothing Design Issues in Military Applications. In Proceedings of the International Conference on Applied Human Factors and Ergonomics; Springer: New York, NY, USA, 24 June 2018; pp. 158–168.
About the authors:
- Sona.M.Anton and Z Shahanazare from the Department of Fashion Design and Arts, Hindustan Institute of Technology and Science, Chennai, Tamil Nadu.
- Dr N Gokarneshan is from the Department of Textile Chemistry, SSM College of Engineering, Komarapalayam, Tamil Nadu.
- Dr U Ratnais from the Department of Textiles and Clothing, Avinashilingam Institute of Home Science and Higher Education of Women, Coimbatore, Tamil Nadu.
- Dr D Anita Rachel is from the Department of Fashion Design, Footwear Design and Development Institute, Noida, Uttar Pradesh.
- Dr C Kayalvizhi is from the Department of Textile Technology, Jaya Engineering College, Tiruninravur, Tamil Nadu
- M Sakthivel and R Devaki are from the Department of Textile Technology, KSR Institute of Technology and Polytechnic College, Tiruchengode, Tamil Nadu.
- S P Thangavel is from the Department of Textile Technology, SSM College of Engineering, Komarapalayam, Tamil Nadu.
- S R Viswanath is from the Department of Textile Technology, Park College of Engineering and Technology, Coimbatore, Tamil Nadu.