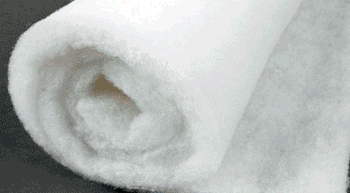
Factors influencing filtration in textile materials
Filtration provides healthier and cleaner products and environment. Textile materials are used in the filtration of air, liquids, in food particles and in industrial production. Filtration fabrics are used widely in vacuum cleaners, power stations, petrochemical plants, sewage disposal, etc. Textile materials, particularly woven and nonwoven are suitable for filtration because of their complicated structure and thickness.
Polyester is widely used in filtration due to its maximum working temperature and very high abrasion resistance, aver Dr P Senthilkumar, Bishwaranjan Ghosh and E Shareen Farzana.
Filtration provides healthier and cleaner products and environment. Textile materials are used in the filtration of air, liquids, in food particles and in industrial production. Filtration fabrics are used widely in vacuum cleaners, power stations, petrochemical plants, sewage disposal, etc. Textile materials, particularly woven and nonwoven are suitable for filtration because of their complicated structure and thickness. Dust particles have to follow a tortuous path around textile fibres. Thus, due to their structure, they have high filtration efficiencies. The usage of the filter fabrics varies according to their end-use. This depends on the properties the filters have which ultimately depends on the characteristics of the raw material used for the manufacturing of the filter fabric.
A filter fabric intended to use for heavy chemical filtration may or may not be used at high temperatures. Similarly, a filter fabric intended to use at the high temperatures may not be a good chemical resistant. Hence, it is totally depending on the type of filter fabric intended to use and the specific end use. In future, filter fabric filtration will play a very critical role in day-to-day life, and there is no single type of fabric being used in all the applications, for the usage of the filter fabrics varies according to their end use.
The current versions of automobiles have an inbuilt filtering system which cannot be used in older vehicles due to structural remodeling. The only way to solve the issue is to prepare an appropriate mix of technical fibres in order to manufacture the best filter fabric that can be easily used by engines of conventional technology that still run on the roads. The main hurdle to such an implementation may be the cost of the fabric manufacturing, which if higher may hinder the wide-spread acceptability. Hence this process is maintained at maintaining a very low production and maintenance costs. By this way this fabric can be easily attached to the device which is attached to the rear end of any exhaust pipe from a diesel vehicle. Moreover it can also be used in industries where there is emission of soot and carbon particles.
The filter can bring noticeable changes to the environment, if the fabric is made to capture carbon and sulphur gases. One remedy to this problem can be by use of catalytic convertor or activated carbon sprinkled between layers of fabric that reacts with sulphur smoke and converts them into non-lethal gases. This needs the use of wide range of fabrics and chemicals to determine the final product useful for filtering gases and soot. There have been many alternate models to capture the soot but they are either very expensive with high maintenance or not efficient enough to bring noticeable changes.
The diesel engines used in automobiles, power plants, generators, etc. are the main contributors of airborne pollution. Although these have become an integral part for any country and the proper technology to curb the pollutants has not been implied on a large scale. It is a well-known fact that no nation can survive without automobiles or power plants, hence finding a middle path to solve the crisis are the priority for any industrial community. The textile fabrics traditionally have not been used for exhaust gas filtration, because of two main reasons. The interstices get chocked up very easily and replenishment or cleaning of the same requires much time. Hence textile filters are limited with industrial and chemical filtration.
Filter medium
Objective of filter medium is to maximize the possibility of collision and the subsequent retention of the suspended particles with fibrous structures while minimizing the energy loss of the system. The efficiency of filtration in industrial fabrics is affected by their porosity. Permeability is capacity of porous materials to transmit the fluids. Liquid and gas permeability increases with the increase in porosity of the fabric. The type of finish also affects the permeability. When Porosity increases pressure drop tends to decrease. When flow rate increases, pressure drop increases. There are various mechanisms by which particles are filtered by filter media. Those are gravitational, impaction, interception, diffusion (Brownian motion) and electrostatic.
There are many different methods of filtration. All aim to attain the separation of substances. Separation is achieved by some form of interaction between the substance or objects to be removed and the filter. The substance that is to pass through the filter must be a fluid, i.e., a liquid or gas. Methods vary depending on the location of the targeted material, i.e., whether it is in the fluid phase or not.
Polyester is widely used in filtration due to its maximum working temperature and it has very high abrasion resistance. Other fibres used in filtration processes are polyaramid, polyimide, cellulosic fibres, silicate fibres, polypropylene, PTFE, polyamide, polyphenylene sulphide, PEEK. All these fibres have maximum working temperature and very good abrasion resistance. They are resistant to acids and mild alkalis. Generally, for filtrations, synthetic fibres are used because of reduced fabric weight, higher strength, easier handling and replacement, easier separation of filter cakes, resistance to rot, higher filtration rate, higher fatigue resistance, good dimensional stability, and high temperature resistance and, better abrasion, corrosion and chemical resistance.
There are basically four types of yarn to be used as filter fabric, namely monofilament, multifilament, fibrillated tape and staple-fibre yarns. Woven fabrics with plain, twill and satin weaves, link fabrics and needle felts are used in filtration applications. .Woven fabric and Needled fabric are both used in fabric filters. The type of fabric construction has closely followed the requirements of mechanism of the fabric filter being used. Typically reverse air fabric filters use woven fabrics, where as mechanical shake/deflate fabric filters use woven fabrics and reverse pulse jet filters use needled fabrics.
Nonwoven materials used in filtration applications are an important part of the worldwide nonwoven industry. Nonwoven filter materials continue to grow and capture a growing share of the filter media market because of their excellent performance and economical cost. It is a large industry, but very diverse with many types of filter producers supplying a wide variety of filtering systems and filter media to meet a wide variety of liquid, air and gaseous fluids filtration needs. The average consumer is aware of filters used in their daily life, such as coffee filters, tea bags, furnace filters and disposable vacuum cleaner bags. They may be unaware of the filter systems and media used for their drinking water, air filtration at their work place or in their car, food and beverage filtration and a host of industrial processes which use filtration to separate materials, reduce pollution and improve the quality of their life. Advantages of nonwoven filter over the woven filters are high permeability, high filtration efficiency, less blinding tendency, no yarn slippage as in woven media, good gasketing characteristics and good cake discharge.
As fibre diameter increases, filtration efficiency decreases. Also, void volume, which is required to reduce the pressure drop, is more in case of nonwoven (98 per cent) than that of woven or knitted (70 per cent), thus nonwovens are advantageous to use.
Traditionally, antistatic woven and non-woven filter media are manufactured by doping polymers with metal salts or carbon material during yarn production or as a separate fibre filament in the yarn preparation stage. However, alloying polyester and other synthetic yarns has the tendency to significantly deteriorate yarn mechanical properties. Polyaniline in its doped form is electrically conductive and is soluble in organic solvents, for example, toluene and xylene. Polyaniline solutions can be used to treat filtration fabrics to improve their dewatering performance. Compared to traditional antistatic filtration fabrics, polyaniline treated polyester fabrics have the advantage of being easily convertible into various kinds of filtration cloths and bags.
Because of the growing use of nonwoven structures in a wide variety of technical applications, the ability to predict specific properties such as permeability is an important phenomenon to understand. The overall permeability of a multilayer heterogeneous nonwoven fabric can be measured by several different testing systems or it can be predicted based on different concepts about the nature of fluid flow. The pressure gradient through a bed of porous material is a function of fluid velocity, fluid and material densities, rate of fluid flow, and material porosity. When considering the flow through porous materials, the shape, arrangement, and size distribution voids through which flow must occur are extremely important. In fact, there is no simple correlation between permeability and porosity because of the strong dependence of flow rate on the width, shape and tortuosity of the conducting channels. Polyanaline in its doped form is electrically conductive and is soluble in organic solvents for example, toluene and xylene.
Polyanaline solutions can be used to treat filtration fabrics to improve their dewatering performance. Tortuosity, the ratio of effective channel length and sample thickness, is an important factor in determining flow through nonwoven materials. Theoretical methods have been developed to understand the relationship between the structure of nonwovens, the properties of fibers used in these structures, and the measurement techniques that are common to property assessment.
Relation between cross-section and filtration
The interstice of the unit cell of the fabric is of too unusual a form to permit a complete hydrodynamic analysis. It is quite possible, however, to define the cross-sectional area of an interstice and determine its minimum value by graphical and, in some case, analytical techniques. In this way, the effect of yarn and fabric structure on minimum pore area can be determined and used to justify empirically determined relationships between cloth design and air permeability.
Figure 1 discusses about the various overlapping pattern for the creation of interstices in a woven fabric. If planes are perpendicular to the fabric surfaces and bisecting any 2 adjacent warps and filling (weft) yarns are used to form a unit cell, four basic categories of pores can be establishes TYPE 1, in which all four yarns of the unit cell alternate form the top to the bottom surface of the cloth and vice-versa; TYPE 2, in which one warp and one filling yarn alternate; TYPE 3, in which no alteration of the yarns takes place; TYPE 4, in which either two warp yarns or two filling yarns alternate from top to bottom surface or vice-versa.
Effect of weave on filtration
Most commercial fabric constructions have two or three types of pores. Figure 2 displays the cross-section of the various types of interstices in a woven fabric.
The plain weave consists entirely of Type I interstice. Few weaves contain all four types.
If two fabrics are woven with identical yarn diameters and yarn spacing?s, they may vary markedly in regard to pore volume and space, depending upon the manner of interlacing of the threads. The weave determines the number of each type of interstice present in a fabric and therefore the general form and cross-sectional area of the pores. The variation of the shape of each of the interstice types, in which progressive horizontal sections of the four pore types have been drawn, much in the same manner that cut longitudinal sections of actual fabrics. It is evident that the interstice with the greatest number of yarn inter lacings, Type I, has the smallest minimum cross-sectional area for the relatively open fabrics considered here, while the pore with no inter lacings, Type III, has the largest minimum cross-sectional area.
On the basis of pore-sectional area, it may be expected, then, that among relatively open fabrics having the same yarn diameters and spacing?s, those with plain weaves will offer most resistance to the passage of gases. Long-float twills or sateen, on the other hand, with the greatest number of Type III interstices, will show the maximum permeability.
The anticipation of a relationship between the horizontal pore area and the air permeability of the fabric assumes, of course, that the smallest effective pore area is parallel to the plane of the fabric. This assumption has no analytical justification, in view of the irregular shapes of the various pore types, but it is accepted as representative of an average condition in order to avoid undue complication in the geometric considerations to follow.
Effect of twist on filtration
The twist direction, as well as magnitude, affects the air permeability of woven fabrics. If the warp and weft twists are both S and both Z twist, the fabric structure will be more compact due to nesting of fibers at points of contact between warp and weft yarns. In twill constructions, a less conspicuous twill line accompanies the combination of S-S or Z-Z twist, and the fabric itself is less porous and has lower air permeability. Conversely, S-Z or Z-S twist results in more pronounced twill, a more open fabric structure, and higher permeability. It can likewise be shown that the direction of the rib of a twill fabric influences its structure and therefore its permeability. The use to which a fabric is put will often change its structure and, at the same time, its permeability. Heavy pressure or calendaring of a fabric during finishing will alter its air permeability. Shrinkage during laundering has a significant effect. Napping or Abrasion will alter structure and permeability.
Finally, tension of the fabric during use will modify its flow characteristics. This is particularly important in the case of parachute fabrics, which must be tested under tension and at air pressures corresponding to flight conditions to enable a satisfactory prediction of the performance of a parachute. This is not a simple matter of stretching the fabric bi-axially and widening the pores. Tension on woven structures results in a change of yarn spacing?s, in crimp interchange, in thread shear, in yarn flattening at crossovers, and in increased yarn densities. All of these factors are related to minimum pore areas.
It is necessary that parachute designers determine the significance of their individual or combined effect in order to predict end-item performance under all possible conditions.
Contribution of inter-fibre pores in filtration
The contribution of inter-fibre pores to the free passage of air through textile fabrics is difficult to measure quantitatively. By blocking inter-fibre pores the discharge coefficient did not change by more than 10 per cent. What effect in blocking the inter-fibre pores may have in the case of higher delivery ratios has not been determined, but it is assumed that in very densely packed fabrics the space between yarns will be of the same order of magnitude as the spacing between fibers in a yarn. In several cases, the inter-yarn pores become apparent only when the materials were placed in front of a strong light and were viewed at an angle to the plane of the cloth surface. However, in the case of the fabrics which were constructed with maximum tightness and did not evidence inter-yarn pore through the fabric, it was noticed that a great deal, more light was transmitted between yarns than through the yarns, as might have been expected from the circularity of the yarn cross sections.
Conclusion
Right kind of filter fabric for the appropriate application can be designed by considering structural parameters such as cross-section, twist in yarn and its direction, weave pattern and inter-fibre pores. Each filter fabric is intended for a specific use in the specific climate under the specific conditions for its optimum output and enhanced efficiency. In future, filter fabric filtration will play a very critical role in our day-to-day life. There is no single type of fabric used in all the applications. The usage of the filter fabrics varies according to their end use. New fibre polymers and fabric finishes are being investigated to address the expanding requirements for fabric filters.
References
- Spiro, T.G.Stigliani, WM (1996), ?Chemistry of the environment? Prentice-Hall ; London.
- HireniMankodi, Pravin Patel, (2009) ?Study on the effect of commingling parameters on glass/polypropylene hybrid yarns properties?., AUTEX Research Journal Vol. No3.
- Turbak, A.F. (1993). ?Nonwovens: Theory, process, performance and testing?. TAPPI Press, Atlanta, GA.
- HireniMankodi, Pravin Patel, (2009) ?New engineering material for textile composites?. Proceedings of the HPTEX.
- Horrock A R and Anand S C, 2000, Handbook of Technical Textiles, Wood head Publication.
- Prof U.J Patil, May 2011 ?Filtration in textile: A review?, The Indian Textile Journal, DKTE? Textile & Engg Institute, Ichalkaranji
- Van Osdell DW, MB Ranade, GP Greiner, and DF Furlong, November 1982, ?Electrostatic Augmentation of Fabric Filtration: Pulse-jet Pilot Unit Experience? (EPA- 600/7-82-062).
- Donovan, R. P., 1985, ?Fabric Filtration For Combustion Sources?, Marcel Dekker Inc, NewYork.
- Turner, JH, and JD McKenna,1984, ?Control of Particles by Filters?, in Handbook of Air Pollution Technology, John Wiley & Sons, New York.
- Palazzo, L., J. Woolston, and P Ristevski, Retrofitting Shaker Baghouses to Cartridge Pulse Jet Technology in The User and Fabric Filtration Equipment – VII, Proceedings, Toronto, September 12 – 14, 1994, Air and Waste Management Association, Pittsburgh.
- Penny, C. W., Electrostatic Effects in Fabric Filtration: Volume 1. Fields, Fabrics, and Particles (Annotated Data), September 1978 (EPA-600/7-78-142A).
- J.Srinivasan and S Katharvelu: Nonwoven Fabric Filtration Media, Synthetic Fibers, April and June 2006.
- Kothari V K, Das A and Sing S: Filtration Behaviour of Woven and Nonwoven Fabrics, IJFTR, June 2007.
- Chatterjee, Bhoumick, Jhalani and Munuja, (TITS Biwani) and Mani (IIT Delhi): Woven Filter Fabric For Controlling Air Pollution, Man-made Textile in India, Nov 1991.
- J sievert and F Loffler, ?Actions to which the filter Medium is subjected in Reverse Jet Bag Filters?, Zement ? Kalk ? Gips, 3 1986 no. 3 71?72.
- A T Purdy, ?The structural mechanics of needle felt filter media?, Second World Filtration Congress, The Filtration Society, Uplands Press, London, 1979, 117?132.
- S C Anand and P J Lawton, ?The development of knitted structures for filtration?, J.Textile Inst., 1991 82(3) 297.
- D B Purchas, ?Practical applications of theory?, Solid/Liquid Separation Technology, Uplands Press, Croydon, 1981, Chapter 10, 595?693.
- A Rushton and P V R Griffiths, in Filtration, Principles and Practices Part I ed C. Orr, Marcel Dekker, New York, 1977, 260.
- A T Purdy, Needle-Punching, Monograph No. 3, The Textile Institute, Manchester, 1980.
- Arun Naik: Textile Filter Media-Part II, ITJ, Feb, 1983.
Dr P Senthilkumar is with the Department of Textile Technology, PSG College of Technology, Coimbatore 641 004. He can be contacted at: Email: senthiltxt11@gmail.com Bishwaranjan Ghosh is with the Sardar Vallabhbhai Patel International School of Textiles & Management, Coimbatore 641 004.